CBE Seminar: Advancing Brain-machine Interfaces, Ultra-compliant Neural Interfaces and Brain-insertion Mechanics
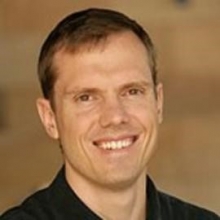
Department of Materials Science and Engineering
Director, Stanford Nanofabrication Facility
Stanford University, Stanford, CA
Abstract: Enabled by new materials and device designs, a new generation of brain interface technologies is replacing bulkier, noncompliant systems with the aim of seamless electronic-biological interfaces with lower tissue damage, reduced immunogenicity, high-density, tunable spatial distribution and long-term stability. Recent successful examples leveraging mechanically compliant materials have demonstrated major breakthroughs in brain research using ultra-flexible systems for ECoGs recordings and for depth electrodes. Yet, surgical implantation damage, scalable channel count and the number of devices implanted simultaneously are still significant challenges.
Here, we apply a materials science approach to understanding how different size probes affect brain tissue when inserted in vivo. Using a modified nano-indenter head, these provide some of the most sensitive force and spatial measurements ever recorded and can be coupled with optical imaging. We discovered a number of unanticipated results, including constant insertion force past pia penetration, existence of a 'no bleeding' size regime, and observed the blood vessel failure mode. We explain these observations based on mechanical models for crack propagation and tissue displacement, and suggest a new framework for understanding tissue trauma during surgery.
In order to take advantage of these new mechanical insights, we developed the Neuroroot platform for facile implantation of ultra-low damage and scalable channel-count penetrating electrodes for chronic brain recording and stimulation. The platform consists of dangling root electrodes only 7 microns wide by 1.5 microns thick, matching both cell size dimensions and tissue mechanical properties, yet without interconnectivity between electrodes that can lead to tissue damage and block nutrient diffusion. We developed a surgical apparatus based on the commonly used NeuraLynx Halo Microdrives to easily and precisely insert these ultraflexible arrays into the tissue target of interest. The microwires are too flexible to insert on their own, thus we developed an ultra slim, 35 micron diameter microwire as a temporary shuttle onto which numerous individual electrodes self-align. Once inserted, these wires delaminate, and the shuttle is removed.
This strategy enables implantation of a number of electrodes into various brain regions at once. Initial chronic implantation of an array of 32 electrodes into the hippocampus of freely moving trained rats exhibit recordings of single unit potentials a few minutes after electrode implantation, as well as a minimal damage both acute and chronic. The open structure of the mesh is believed to minimally perturb the ecosystem and nutriment diffusion. Subsequent implantation into the medial entorhinal cortex of trained rats has demonstrated the ability of this platform to be implanted into regions that are difficult to access for traditional probes.
Bio: Nicholas Melosh received his bachelor's degree in chemistry from Harvey Mudd College in 1996, then went on for a doctorate in materials science at UC Santa Barbara working with Brad Chmelka, Galen Stucky and Glenn Fredrickson. He worked with Professor Jim Heath at UCLA/Caltech as a postdoc from 2001-2003 and joined the Department of Materials Science and Engineering at Stanford University in 2003. Melosh's interests include interfacing inorganic structures with biology, brain-machine interfaces and plasmonics. He is a Terman Fellow and Reid and Polly Anderson Faculty Scholar at Stanford University.