UCI Engineers Collaborate to Attack Virus
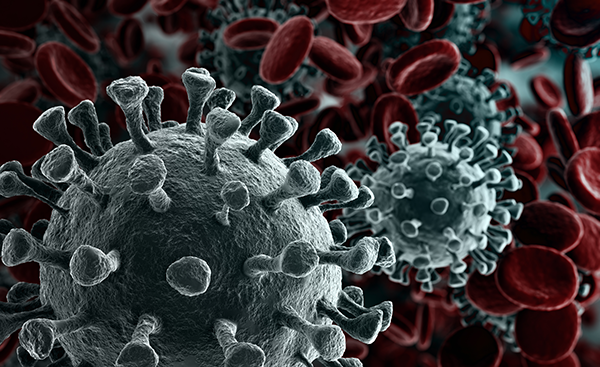
The coronavirus outbreak is causing researchers around the world to launch myriad investigations. Engineers at the Samueli School are among those searching for answers and proposing solutions to the grand challenge posed by the COVID-19 pandemic.
April 10, 2020 - As devastating as the coronavirus has been worldwide, the pandemic has ignited a can-do spirit in the Samueli School of Engineering. Faculty, graduate students and staff researchers are rolling up their sleeves, working in labs, homes and garages in an effort to help. From diagnostic strips to makeshift ventilators to antibody detection techniques, engineers are collaborating across disciplines to create devices and treatments that they hope can mitigate the crisis.
Samueli School Dean Gregory Washington applauds the selfless efforts of his faculty and staff during a time of crisis. “It’s heartwarming to see the number of faculty involved in COVID-19 related research, essentially without a guarantee of funding, but because they see a need to address a grand challenge problem and make solutions happen.”
Here are some of the ongoing projects at the Samueli School:
Jump to:
- Ventilators
- Coronavirus (SARS-CoV-2) Paper Strip Self-test
- Antibody Detection
- Virus Mutation
- Virus Transmission
Ventilators
COVID-19 often attacks the lungs, requiring patients with the most serious symptoms to use ventilators to breathe while their bodies fight the virus. Unfortunately, a shortage of medical ventilators has endangered or taken the lives of thousands of these patients.
Samueli School engineers are answering the call for simple and affordable ventilators, using off-the-shelf parts to meet the unprecedented demand. Many are participating in the Bridge Ventilator Consortium, a team consisting of physicians, engineers and biomedical device experts. They meet daily via Zoom, to collaborate on building devices for patients who need help breathing but don’t require the top-of-the-line ventilators that are currently in such short supply.
The consortium is led by Dr. Brian Wong, a UC Irvine facial plastic surgeon who has a joint appointment in biomedical engineering and at the Beckman Laser Institute and Medical Center (BLI). Partners in the effort include Virgin Orbit, a rocket manufacturer who is turning its Long Beach factory into a ventilator manufacturer, and University of Texas, Austin. The collaborators are working to address the equipment shortages with open source designs.
“The Bridge Ventilator Consortium is a think tank, incubator, idea forum, engineering fight club and venture capital forum, among many other things,” Wong said. “It brings people together to move fast.”
Different teams are taking different approaches to these “bridge” ventilators. Some take their design from the handheld “ambu” bags used by paramedics to deliver oxygen over short periods of time. A prototype conceived by BLI’s Tom Milner, who also has a lab at UT Austin, is driven by a windshield wiper-type motor, which swings a wheel to compress the bag. Elliot Hui, biomedical engineering associate professor, along with his graduate students Vincent Zaballa and Erik Werner, are duplicating this prototype in his UCI lab. Undergraduate mechanical engineering student Luis Ramirez is contributing to the effort from home with his 3D printer. “The idea behind our approach is to replicate the thumb compression that the ambu bag is designed for,” Hui said, explaining that the swinging motion of the motor can be electronically modulated to change critical variables including tidal volume, respiratory rate and inspiration-to-expiration ratio, so it is easily adaptable to specific patient needs.
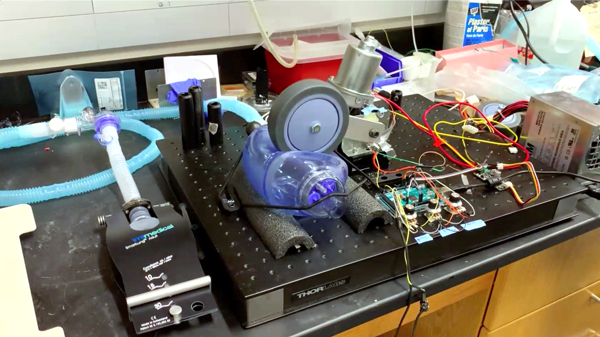
The motor putting pressure on the ambu bag ventilator in Elliot Hui’s lab replicates the thumb compression that the bag was designed for.
These ventilators could sell for under $500, compared to as much as $30,000 for top-of-the-line ventilators. “This one is going to basically be for all the patients who need a ventilator but do not need a top-line ventilator,” said Dr. Govind Rajan, director of clinical affairs at UCI Medical Center and a co-leader of the consortium.
The group is in the process of obtaining FDA emergency use approval for its design.
Marc Madou, Chancellor’s Professor of mechanical engineering, and his BioMEMS team are using a pneumatic approach on their device. Called the Ventivader, the ventilator uses a pressurized chamber to send compressed air through inhalation and exhalation valves into and out of a patient’s lungs. Controlled by low-cost electronics, including a pressure sensor and a Raspberry Pi controller, the device is simple in design and can be built inexpensively, according to Madou. Team members include researcher Horatio Kido, Sasha Perebikovsky, who recently earned her Ph.D., and doctoral candidate Ehsan Shamloo Aliabadi. See video here.
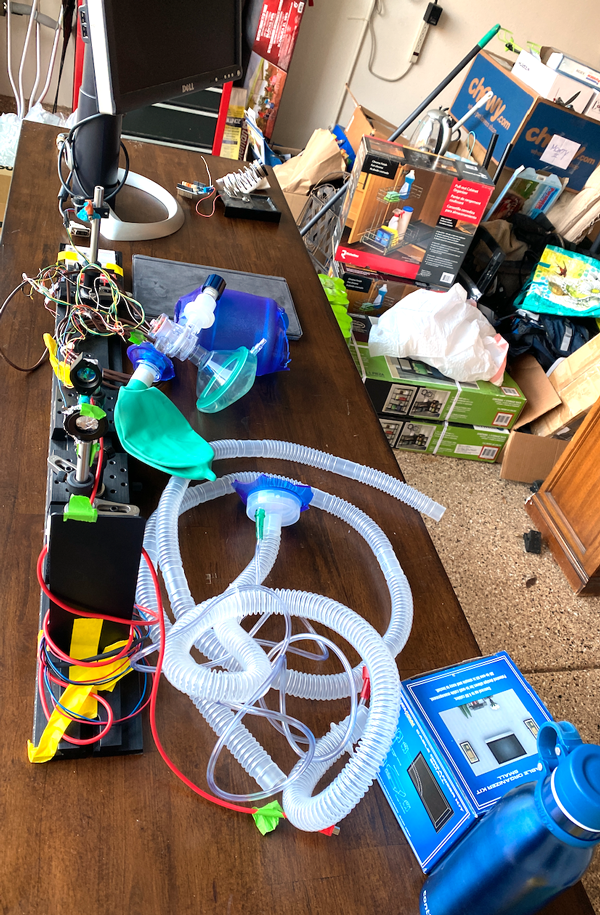
The ventilator prototype designed by Botvinick, Choi and their BLI colleagues utilizes a modified CPAP device.
Elliot Botvinick and Bernard Choi, biomedical engineering professors and professors of surgery at BLI, are collaborating with BLI colleagues on a ventilator that repurposes a common household device, a Continuous Positive Air Pressure (CPAP) machine used in the treatment of sleep apnea. See video here.
“There is an abundance of available CPAP devices because users typically upgrade their device every few years, keeping the old one in storage. These abandoned devices can be donated and repurposed for medical ventilation − with proper engineering and medical guidance,” said Botvinick.
Dr. Matt Brenner, professor of medicine and a pulmonologist who is BLI’s interim director, proposed the idea; Botvinick and Choi are leading design efforts. “CPAP devices are very commonly used and provide pressure to keep airways open. They can be modified to provide increased pressure, and with further design enhancements proposed by Professors Botvinick and Choi, can provide intermittent positive pressure breaths,” said Brenner.
Potential benefits include the ability of the patient to control his/her own breathing rate (with a backup rate available if needed), and the fact that pressure-limited breaths may cause less trauma to the lungs than volume-based ventilator breaths. “Many of the important safety features and sensors are already present in CPAP devices, which can help reduce costs as well as improve safety,” Brenner said.
Yet another low-cost medical ventilator design that can be customized for each patient’s needs is underway in Haithem Taha’s Aeronautics Dynamics and Control Lab. Taha, mechanical engineering assistant professor, and his doctoral student Moatasem Fouda, with the guidance of two medical doctors, developed a design that replaces several valves with a simple unit called a Respiratory Regulation Unit (RRU). See video here.
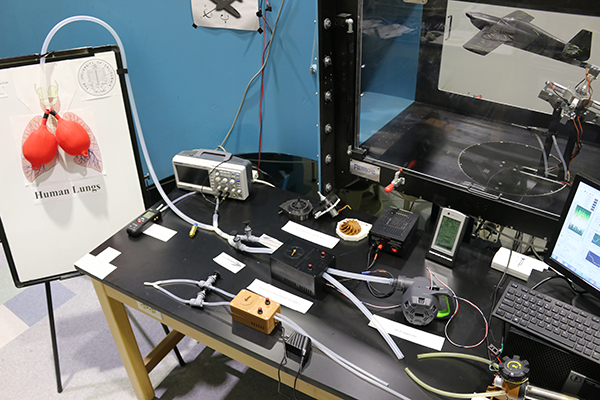
The ventilator under development in Taha’s lab utilizes a mechanically controlled respiratory regulation device connected to a high-pressure air supply. These ventilators have stand-alone functionality so they can easily be deployed in field hospitals.
The RRU is connected to a high-pressure air supply that passes compressed air to the patient through tubing. The process is controlled mechanically, without the use of electronics, and is monitored by an attached pressure gauge. The current model uses a commercially available air compressor, while future iterations will employ a proprietary compressor design inspired by jet engines, which blends oxygen and air. "Unlike the ambo bag squeezer, this RRU allows wider control of ventilator operations, including lung pressure and volume, respiration rates and inhale/exhale ratios," Taha said.
He believes it is possible to manufacture 10,000 of these units in one to two weeks. Because they can function independently, without being connected to a separate high-pressure air source, they can be used in field hospitals, like the one in New York’s Central Park.
Bridge Ventilator Consortium founder Brian Wong summed up the many efforts underway. “So many engineers, so many companies, and all give selflessly. We stand on each others’ shoulders.”
Coronavirus (SARS-CoV-2) Paper Strip Self-test
Widespread, accurate testing for COVID-19 infection is critical for informing people’s behavior, delivering appropriate care and tracking the virus’s spread. Currently, testing involves collecting a sample from the nose and/or throat with a special swab at a designated collection location. This process is painful, slow and requires a health care professional. Samples are sent to a clinical lab and patients wait, from several hours to a week, for results from a Polymerase Chain Reaction (PCR) assay. The sample collection method requires close contact between health care workers and patients, posing risk of transmission, and with limited swabs and PPE equipment, can be logistically challenging.
Over the last few weeks, much simpler paper-strip assays, which target a patient’s immune response to immediate or prolonged exposure to the coronavirus, have begun to emerge. These tests require a blood sample collection, need further sample preparation and treatment, and are designed to be performed in clinical settings. Moreover, the accuracy of these tests has been called into question lately.
An engineering-led team of UCI researchers is developing a rapid, direct test for the SARS-CoV-2 virus itself, as opposed to the infection caused by the virus. Such a rapid immuno-assay could be widely distributed to medical personnel, clinics, hospitals and the public. The team is designing a simple, lateral flow assay (based on a paper strip), similar to a home pregnancy test, that could be sent to people’s homes for self-screening of the general population. Medical workers could be among the first targeted recipients of such a test to determine whether they are uninfected when leaving the hospital.
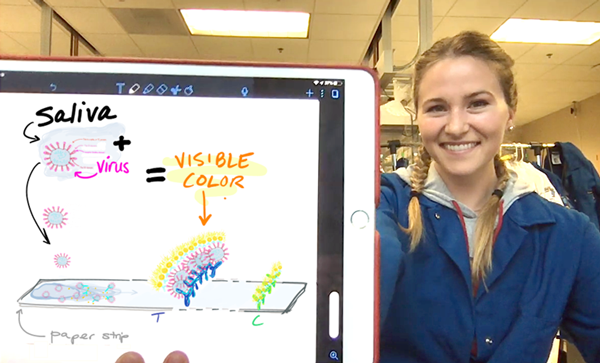
Julia Zakashansky, materials science and engineering doctoral student, spearheads the CoronaStrip effort in the Khine lab, coordinating a team of students participating both in-person and remotely: from Orange County to Seattle to Sao Paolo, Brazil. “Our lateral flow assay is extremely simple and will provide a color-change result if the virus is detected in saliva samples,” explains Zakashansky.
“Saliva has been shown to have a better than 90% correlation with nasopharyngeal specimens in detecting respiratory viruses, including coronaviruses,” said Michelle Khine, professor of biomedical engineering and project PI.
Most importantly, these test strips will be matched to a cell phone app that automatically images and uploads the results for anonymous tracking of the location (randomized over a 1-mile radius to ensure HIPAA protection), generating heat maps of infection zones and allowing for real-time epidemiological surveillance.
If the test is positive, the individual would be instructed on follow-up testing and medical attention. This would free up critical health care personnel by providing an at-home prescreen as well as a quick diagnostic for health care providers themselves, who must know if they are infected.
The “CoronaStrip” will be tested in the UCI Department of Emergency Medicine. In addition to Khine, Professor Elliot Botvinick, biomedical engineering, and Chancellor’s Professor Plamen Atanassov, chemical and biomolecular engineering, are collaborating with colleagues from emergency medicine, including Associate Professor Sean Young and Professor Shahram Lotfipour, M.D.
Antibody Detection
The human toll is far and away the most serious consequence of COVID-19, but the economy has suffered a blow as well. The key to getting people back to work could lie in determining who might be immune to the virus. Those people could return to school and work, helping to slowly restart the economy. Two Samueli School professors are working to develop antibody tests to help identify those with immunity.
Peter Burke, professor of electrical engineering, is proposing an inexpensive point-of-care method to detect COVID-19 immunity. (Burke and colleagues at the University of Illinois have recently filed for a patent.) Antibodies in blood serum presumably can be used to determine immune patients. Ordinarily, blood tests need expensive proteins to detect antibodies produced by the immune system in response to infection, but Burke is investigating the feasibility of using short DNA sequences as the capture agent instead. He says this could be less expensive to manufacture, and could even be implemented on a low-cost paper test, like those used for pregnancy. Those who test positive for the antibodies would presumably no longer be infectious and could be cleared to return to work. Low-cost tests such as Burke’s would enable clinicians to determine how long this immunity lasts and how robust the immunity is at a global population level.
Burke says the primary goal of his study is to find and exploit DNA- or RNA-aptamers (short strands of DNA) that bind with specific affinity to the binding site of antibodies for the virus. These are known to be specific to the virus’s spike protein. “If we are successful, then a point-of-care, in-home test of patients that are immune to COVID-19 could follow,” Burke said, adding that it could be paper-based and cost only a few cents to produce. “This would be like a pregnancy test for COVID-19 immune patients.”
Marc Madou also is working on antibody testing. He is collaborating with immunologist Phil Felgner, director of UCI’s Vaccine Research and Development Center and the Protein Microarray Laboratory and Training Facility, to develop a CD system that can provide low-cost, high-throughput semi-automated immunoassay processing.
Over the past 15 years, Felgner’s laboratory has developed a protein microarray analysis that can quantify antigen-specific antibody responses induced after infection from any microorganism. Felgner and colleagues recently developed a coronavirus antigen microarray that includes multiple antigens from each of the four common coronavirus strains, as well as from the SARS, MERS and SARS-CoV-2 emerging coronavirus strains. The protein microarray also includes antigens from other common respiratory viruses including influenza, which will generate additional data and serve as a control for coronavirus infection.
Madou’s team pioneered a CD fluidic platform that has now been commercialized by several companies for different applications.
Together, the two are working to integrate coronavirus antigen arrays onto CD-based fluidic platforms, which could lead to a quick, inexpensive microarray digital imaging test. The key innovation in the disposable immunoassay disc design is a fluidic structure that enables efficient micro-mixing. “Several arrays can be put on the CD at once and the spinning makes the binding of the proteins go 10 times faster,” Madou said.
He added that the system is simpler than his previous fluidic CD systems for three reasons: blood samples need no sample preparation; the system uses fluorescence to indicate protein binding, a much quicker approach than absorption; and newly available digital fluorescence microscopes reduce assay quantification costs.
Data processing of results will be cloud-based, allowing for rapid analysis – Madou expects about 10 minutes.
“This platform is particularly relevant today with the coronavirus outbreak because of the urgent need to understand who in our environment has been exposed to the virus, to predict whether those people are susceptible to severe, mild or asymptomatic infection, or whether they have protective antibodies and are unknowingly spreading the infection to close contacts,” he said.
Virus Mutation
While much of the country is worried about flattening the curve through social distancing, bioengineers are preparing to deal not just with the current wave of the coronavirus but future waves and outbreaks. They want to help researchers and public health officials track and protect against the spread of the virus over time as it mutates.
Biomedical engineer Chang Liu is applying his expertise in genetic engineering and directed evolution to help find potential therapeutic and diagnostic agents for SARS-CoV-2, the virus responsible for the COVID-19 pandemic. Liu’s lab has developed a technique called orthogonal DNA replication, which is capable of continuous hypermutation of genes in an engineered yeast cell. He is using this synthetic genetic system to detect and neutralize a key interaction between the coronavirus’s spike protein and the cellular receptor, ACE2, which acts as coronavirus’ main entry point into the cell.
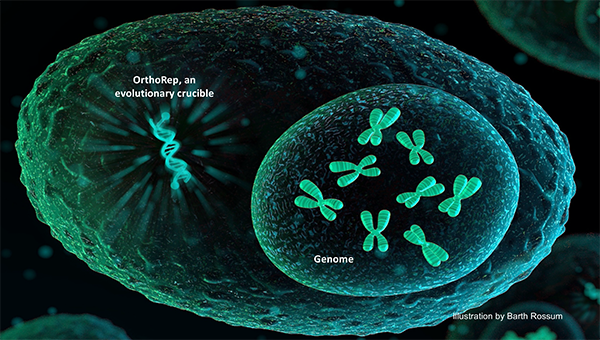
Chang Liu’s orthogonal DNA replication system is capable of continuous hypermutation of genes. He is using it to develop a protein evolution system that can mimic the immune system and find answers about the coronavirus.
“We are working on an accelerated protein evolution system that can mimic natural immune systems, but that works on a faster pace and is primed for generating high-affinity binding proteins against SARS-CoV-2 and new versions of the virus should they evolve,” said Liu, assistant professor of biomedical engineering. “We will use this system to evolve high-quality nanobodies and ACE2 variants to detect and neutralize the SARS-CoV-2 through binding of its spike protein.”
Such a process could be crucial to fighting current and future waves of the coronavirus pandemic. With this rapid evolution system, researchers will be ready to generate binding proteins against SARS-CoV-2 and future coronaviruses within two weeks of obtaining a target. Quickly evolved high-quality binding proteins can act as critical reagents in detection platforms and as therapeutic candidates for the protection of medical workers before patient-derived antibodies, conventional therapeutics and vaccines are available.
UCI postdoctoral researcher Alon Wellner and graduate student Ming Han Ho are working with Liu on this project, as are biology colleagues Andrew Kruse and Conor McMahon from Harvard Medical School.
Virus Transmission
To date, the U.S. Centers for Disease Control and Prevention and other health agencies have believed the coronavirus’s primary route of transmission is through large respiratory droplets emitted in a cough or sneeze. But transmission is not fully understood, and scientists are debating whether the novel coronavirus can spread through the air.
UCI civil engineering and environmental researchers are investigating the risks of exposure to the virus through contaminated aerosols in the bathroom. According to Sunny Jiang, professor of civil and environmental engineering, researchers in Wuhan, China, detected live SARS-CoV-2 in patients’ feces, raising concerns of possible transmission through aerosols generated from the toilet or drains connected with the building sewer pipe in multi-unit buildings, such as apartments or hospitals.
Her team conducted a quantitative microbial risk assessment to estimate the health risks associated with two aerosol exposure scenarios: toilet flushing and faulty drainage/sewage plumbing. SARS-CoV-2 data were collected from the rapidly emerging literature. The human exposure dose was calculated using a simulation of viral concentrations in aerosols under each scenario and human breathing rates.
Jiang says the results indicate the median risks of developing COVID-19 are generally low for both scenarios. However, the worst-case scenario from using a documented SARS-CoV-2 concentration in aerosols of hospital toilet rooms showed a risk of over one in 1,000 chance. The research has been submitted for publication in Environmental Science and Technology. Jiang and graduate student Hunter Quon worked with environmental researchers Kuangwei Shi, Zi-Lu Ou-Yang and Chengwen Wang from Tsinghua University in Beijing China.
– Anna Lynn Sptizer and Lori Brandt