MSE 298 Seminar: Advancements in Vertical GaN p-n Junction Structures Via P-type Ion Implantation and High Quality Substrates
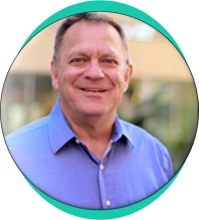
Professor
Department of Materials Science and Engineering
UCLA
Abstract: Vertical GaN power devices have emerged to become promising candidates for next-generation high power applications due to superior material properties such as high breakdown voltage, low on-resistance, and high mobility compared to devices based on Si and SiC. GaN-based p-n junction switching devices enable higher voltage power with significantly higher efficiencies with added advantages of systems with reduced size and weight. A technological limitation of GaN, however, has been the inability to achieve high p-type doping in a planar, vertical device. Here, we will focus on recent developments to achieve high p-type efficiency though ion implantation, novel high temperature annealing schemes, and the importance of defects and morphology in native substrates and epitaxial layers.
GaN epitaxial layers for subsequent p-type ion implantation can be grown on sapphire substrates but vertical devices are optimal when using GaN homoepitaxial structures. Unlike most other semiconductor substrates, GaN substrates are not grown from the melt. Hydride vapor phase epitaxy or ammonothermal growth are predominantly used to produce substrates, and these substrates will experience higher temperatures during subsequent epitaxy and ion implant activation annealing than occurs during substrate formation. In both types of substrates, the threading dislocation distribution can vary by orders of magnitude – these variations correlate well with leakage currents in vertical devices. We address the impact of substrate defects on vertical GaN device leakage behavior. In this work, the effects of the substrate defects on the reverse leakage behavior of vertical GaN Schottky and p-i-n diodes are investigated. A direct connection between the reverse leakage behavior of GaN based vertical devices and the dislocation distribution in the substrate was determined. The difference in the leakage current can be as high as 6 orders of magnitude for vertical GaN p-i-n diodes at -200V, using HVPE substrate with inhomogeneous defect distributions (with dot-cores). For comparison, using HVPE substrate with uniform defect distribution (no cores), the p-i-n diodes show much more uniform leakage behavior at -200V, varying within only an order of magnitude. The wafers with inhomogeneous defect distribution possess periodically patterned core centers with higher defect density (up to 108 cm-2) than regions in between cores, which have very low defect concentrations (as low as 104 cm-2). In comparison, wafers without the periodic cores have a uniform defect density (~106 cm-2) and show a small variation in the rocking curve FW0.01M, ranging only from 70 to 280 arcsec. For Schottky diodes fabricated on substrate with cores, positioning the devices away from the core centers results in a reduction of the reverse bias leakage by 2-4 orders of magnitude at -10 V. Similar trends are also observed in the p-i-n diodes, with device further away from the core centers showing lower leakage. The devices in the low defect concentration region (> 200 um away from core centers) on the wafer with cores outperform the devices from the wafer without cores, showing up to 2 orders of magnitude lower leakage current at -200 V. The results from this study show that the substrate defect density and distribution play an important role on the device leakage current and avoiding highly defective regions on the substrate will improve the performance of vertical GaN devices.
Bio: Mark Goorsky is a leader in wafer bonding, layer exfoliation and transfer, and chemical mechanical polishing of semiconductors and optical materials. Goorsky also provides expertise in materials characterization of semiconductor materials and devices, with emphasis on structural (X-ray scattering and electron microscopy) and chemical (electron energy loss spectroscopy, energy dispersive elemental analysis) techniques. His current research areas include materials integration of wide bandgap semiconductors and thin film electro-optic materials such as lithium niobate. He received the universitywide 2016 UCLA Distinguished Teaching Award and the Harvey L. Eby Award for the “Art of Teaching." He was a member (2011-2015) of the U.S. Air Force Science Advisory Board, associate editor (2002-2019) for the Journal of Crystal Growth, a recipeint of the T.S. Walton Award from the Science Foundation of Ireland in 2010 (where he participated in projects to understand the integration of germanium and III-Vs with silicon) and a recipient (1995-2000) of a National Science Foundation CAREER AWARD. He has been on the faculty at UCLA since 1991. He served as department chair from 2005-2010 and on the universitywide Undergraduate Council from 2018-2021.